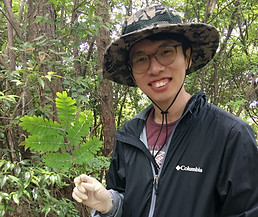
I am an ecologist broadly interested in understanding the causes and consequences of biodiversity.
Ecological and evolutionary processes jointly shape biodiversity. Research in the past decade has recognized the importance of eco-evolutionary interactions for individual populations. However, little is known about how these processes interact in a community context. I incorporate ideas from community ecology, such as the temporal and spatial niche dynamics theory, modern coexistence theory, competition-relatedness hypothesis, and stress-gradient hypothesis, into evolutionary ecology to explore eco-evolutionary interactions at the community level.
​
My research addresses three critical questions. First, how do ecological factors drive adaptive radiation? Second, how does rapid evolution feedback to affect ecological processes? Third, how do the eco-evolutionary interactions together influence species’ responses to environmental changes? I mostly work with microbial communities, including freshwater microbes, aquatic plants’ microbiomes, and model organisms.
​
My main study system is aquatic floating plants and the associated microbial communities. I currently examine the influences of eco-evolutionary interactions within the microbiome on plant hosts.
​
I am recruiting two graduate students, starting in fall 2021. I encourage my students to be independent and develop research projects to complement my research. Internal funds, such as fellowships, teaching assistantships, and research assistantships, are available. I am also happy to assist prospective graduate students with applying for external fellowships, such as the NSF GRFP. If you are interested in any of the research fronts listed below, please feel free to contact me.
1. Using experiments, analysis of existing datasets, and mathematical modeling to understand the ecological and evolutionary mechanisms that direct community assembly and ecosystem functions.
2. Exploring the eco-evolutionary dynamics in plant microbiomes to predict microbial impacts on plant fitness.
3. Exploring the eco-evolutionary responses of plant-microbiome systems to emerging environmental stressors.
​
I will soon recruit one research technician and one postdoctoral researcher. Inquires on these opportunities are also welcomed.
Research
Species phylogenetic relationships and community processes
Phylogeny describes the evolutionary relationships between species. As suggested by Charles Darwin, closely related species may be more similar in traits. These species may require more similar resources and compete more strongly with each other (i.e., Darwin’s relatedness-competition hypothesis). Phylogenetic distance between competing species, therefore, can be used as a predictor for the trait similarity and competitive strength between related species. Based on this idea, I have been testing the explanatory power of phylogenetic distance for community assembly, invasibility, and ecosystem functioning.
Ecological drivers of adaptive evolution
Adaptive radiation is an important evolutionary process, through which a single species lineage diversifies rapidly to utilize various niches within a habitat. I am interested in exploring ecological mechanisms that drive adaptive radiation.
Pseudomonas fluorescens is a common environmental bacterium, widespread in freshwater, soil ecosystems, and plant microbiomes. I use the strain SBW25 as the model of adaptive radiation. The ancestral phenotype of SBW25, smooth morph, rapidly evolves biofilm-forming phenotypes, including wrinkly and fuzzy spreaders in static microcosms. Other members of freshwater communities, such as bacteria, protists, bacteriophages, have been used in my study as the competitors, predators, and parasites of SBW25.
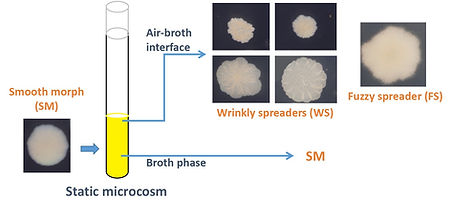
Adaptive radiation of Pseudomonas fluorescens SBW25
Ecological factors can influence adaptive radiation, either through affecting the population size of diversifying lineages or by modifying niche availability for the establishment of newly emerged mutants. My research has shown that temporal and spatial fluctuations in environmental conditions, which constitute temporal and spatial niches, promote biodiversity during adaptive radiation. Meanwhile, the presence of competitors, predators, and parasites reduces the population size of diversifying species, and thus, suppresses their adaptive radiation.

Smooth morph and wrinkly spreader phenotypes colonizing an agar plate
Eco-evolutionary dynamics in plant-microbiome interactions
My current research focuses on the influences of rapid microbial evolution on microbiome community structure and plant host fitness.
​
Traditionally, evolution was thought to occur slowly and impact ecology only over the long term. This is clearly not the case for microorganisms with large effective population size and high mutation rate. I establish the novel experimental system that involves aquatic plants (duckweed Lemna minor and Spirodella polyrhiza) and their microbiomes to examine the role of rapid evolution in plant-microbiome interactions.
​
I found that rapid biofilm evolution modifies microbiome community structure and determine the effect of microbiomes on duckweed fitness. Such an eco-evolutionary interaction happens through the modification of the production of indole-3-acetic acid, a plant growth-promoting hormone produced by the microbiome (Tan et al. in review).
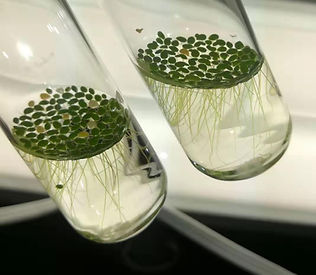
Duckweed Lemna minor interacting with its microbiome in experimental microcosms
Exploring species eco-evolutionary responses to artificially engineered nanoparticles
I recently started a new project, testing the long-term eco-evolutionary impacts of copper oxide nanoparticles (CuO NPs) on yeast. Artificially engineered nanomaterials have become an indispensable component of modern life, and CuO NPs are the materials that have been applied in almost all electronic devices and eventually released into the environment.
​
Microorganisms may develop unique, heritable traits in response to such novel environmental stressors. One possible trait is multicellularity, which reduces the surface-to-volume ratio of individuals and minimizes the impacts of the adverse environment.
​
In the interdisciplinary project, we show that copper oxide nanoparticles promote the evolution of multicellularity in Baker’s yeast in 280 generations. We further conducted mutation and transcriptomic analysis to understand the mechanisms of multicellularity evolution. The multicellular yeast, most of which carry mutations in the transcription factor ACE2, is found under oxidative stress. By downregulating genes coded for copper transportation proteins and upregulating those for aerobic respiration, the multicellular yeast maintains the copper homeostasis and removes the reactive oxygen species within the cells. These mechanisms together allow multicellularity to mitigate nano-induced oxidative stress in yeast cells.
​
I am currently working on the influences of nanoparticles on plant-microbiome interactions. I am testing how the plant ecological and evolutionary dynamics influence the responses of microbiomes (both in community structure and ecosystem functions) to nano stress.

Top left: Copper oxide nanoparticles under a transmission electron microscope.
Top right: A multicellularity yeast cluster under an optical microscope.
Bottom: The dynamics of average cluster size of yeast indicate that larger multicellular yeast evolves in the presence of copper oxide nanoparticles.
Publications
Yang, X.*, J. Tan*, K. H. Sun, and L. Jiang. 2020.
Oecologia, 193:437-447. (*Authors contributed equally)
​
​
Tan, J., X. Yang, Q, He, X. Hua, and L. Jiang. 2020.
Earlier parasite arrival reduced the repeatability of host adaptive radiation.
ISME Journal, 14:2358-2360.
​
​
Wan, N.-F., X.-R. Zheng, L.-W. Fu, L. P. Kiær, Z. Zhang, R. Chaplin-Kramer, M. Dainese, J. Tan, S.-Y. Qiu, Y.-Q. Hu, W.-D. Tian, M. Nie, R.-T. Ju, J-Y. Deng, J.-X. Jiang, Y.-M. Cai & B. Li. 2020.
Global synthesis of effects of plant species diversity on trophic groups and interactions.
Nature Plants, 6:503-510.
​
​
Tan, J.*, Q. He*, J. P. Pentz, C. Peng, X. Yang, M.-H. Tsai, Y. Chen, W. C. Ratcliff, and L. Jiang. 2019.
Nanoparticles promote the evolution of multicellularity in yeast.
Nanotoxicology, 13:597-605. (*Authors contributed equally)
Li, S.-P.*, J. Tan*, X. Yang, C. Ma, and L. Jiang. 2019.
ISME Journal, 13:402–412. (*Authors contributed equally)
​
Yang, X., Z. Yang, J. Tan, G. Li, S. Wan, and L. Jiang. 2018.
Journal of Ecology, 106:991-1000.
Tan, J., J. B. Rattray, X. Yang, and L. Jiang. 2017.
Spatial storage effect promotes biodiversity during adaptive radiation.
Proceedings of the Royal Society of London B, 284:20170841.
Tan, J., X. Yang, and L. Jiang. 2017.
Evolution, 71:1719-1727.
(Cover article. Featured by the Georgia Tech News and the National Science Foundation.)
Ma, C., S. Li, Z. Pu, J. Tan, M. Liu, J. Zhou, H. Li, and L. Jiang. 2016.
Proceedings of the Royal Society of London B, 283:20160663.
Tan, J., M. R. Slattery, X. Yang, and L. Jiang. 2016.
Phylogenetic context determines the role of competition in adaptive radiation.
Proceedings of the Royal Society of London B, 283:20160241.
Tan, J., Z. Pu., W. A. Ryberg, and L. Jiang. 2015.
American Naturalist, 186:59-71.
Pu, Z., P. Daya, J. Tan, and L. Jiang. 2014.
Phylogenetic diversity stabilizes community biomass.
Journal of Plant Ecology, 7:176-187.
Tan, J., C. K. Kelly, and L. Jiang. 2013.
Temporal niche promotes biodiversity during adaptive radiation.
Nature Communications 4, doi:10.1038/ncomms3102.
(Featured by the Georgia Tech Research Horizon)
Tan, J., Z. Pu, W. A. Ryberg, and L. Jiang. 2012.
Species phylogenetic relatedness, priority effects, and ecosystem functioning.
Ecology, 93:1164-1172.
(Recommended by the Faculty of 1000)
​
Jiang, L., L. Brady, and J. Tan. 2011.
Species diversity, invasion, and alternative community states in sequentially assembled communities.
American Naturalist, 178:411-418.
Jiang, L., J. Tan, and Z. Pu. 2010.
An experimental test of Darwin's naturalization hypothesis.
American Naturalist, 175:415-423.
(Recommended by the Faculty of 1000)